Researchers at the Karlsruhe Institute of Technology have advanced mechanical metamaterials to enhance energy storage and efficiency, with potential applications in robotics and industrial machinery, promising significant improvements in sustainability and resource efficiency.
Researchers at the Karlsruhe Institute of Technology (KIT), led by Professor Peter Gumbsch, have made significant advancements in the field of mechanical metamaterials, demonstrating remarkable properties for energy storage and management. These breakthroughs are expected to transform energy efficiency across various applications, including robotics and industrial machinery.
The essence of this research revolves around the concept of enthalpy, which defines the maximum energy density a material can store and subsequently release. Traditional materials often struggle to balance essential characteristics such as stiffness, strength, and recoverable strain, thus limiting their energy storage capabilities. Professor Gumbsch highlighted the necessity of integrating these often conflicting attributes to forge a new category of materials capable of enduring mechanical loads while also maintaining flexibility.
Focusing on a unique helical deformation mechanism in specifically arranged rods, the research team developed a novel metamaterial that effectively captures and retains elastic energy. This approach diverges significantly from conventional bending springs, which frequently face high tensile and compressive stresses that can lead to structural failure. By exploiting the twisting motion inherent to the helical structure, the researchers significantly lowered internal stress levels, enhancing the material’s ability to withstand greater loads without permanent deformation.
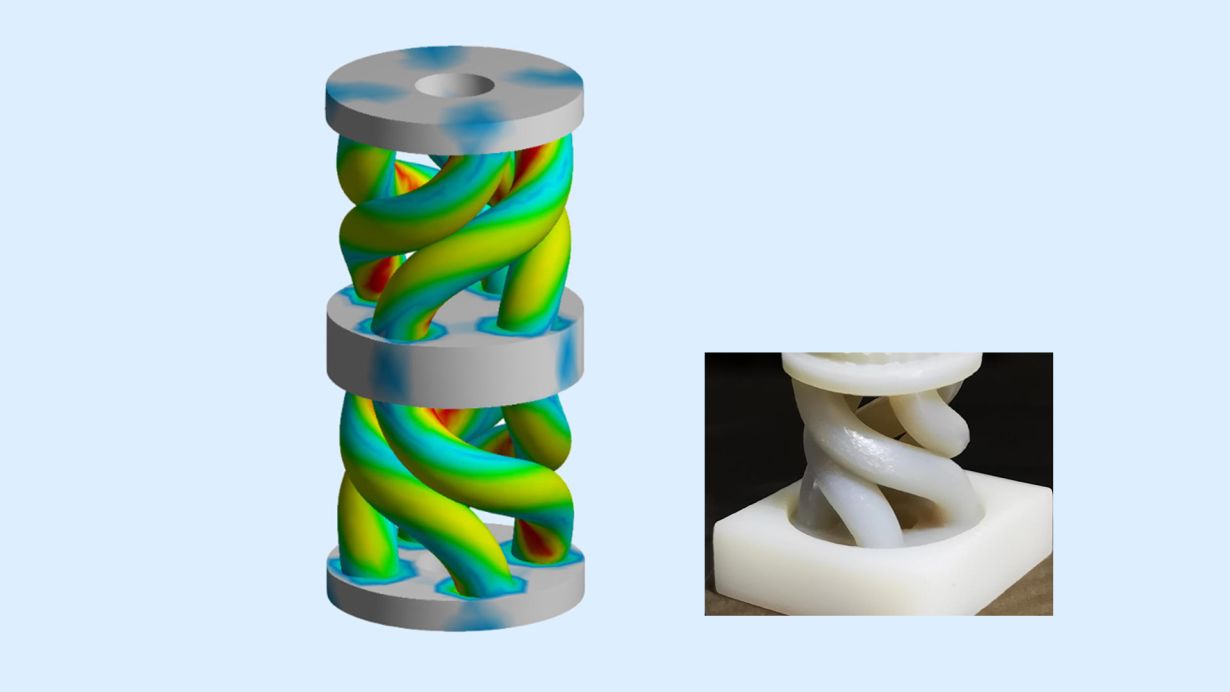
The model shows the helical deformation of the metamaterial. Thanks to this mechanism, storing a high amount of energy is possible without breakages. (Illustrations: IAM, KIT / Collage: Anja Sefrin, KIT)
In their experimental work, Gumbsch and his colleagues conducted simulations that illustrated the unique resilience of this metamaterial under uniaxial loads. Their findings revealed that the material’s enthalpy could reach levels two to 160 times greater than that of existing metamaterials. This dramatic increase in performance presents a significant advantage for engineers and designers focused on improving energy efficiency in a variety of applications.
Rigorous testing confirmed the theoretical models, demonstrating that these metamaterials could absorb substantial forces while maintaining impressive elastic energy storage capabilities. The potential uses of such materials span energy-efficient machinery, robotics, and shock absorption systems, all of which stand to benefit greatly from improved mechanical properties coupled with efficient energy storage.
The metamaterial presents a superior alternative to conventional springs and shock absorbers, with structural properties that may facilitate more compact design while maintaining maximum flexibility and resilience. This innovation is particularly promising for the field of robotics, potentially leading to more agile and responsive robotic systems that leverage advanced mechanical efficiencies.
Beyond conventional applications, the researchers anticipate the potential to use the internal twists and turns of these metamaterials to create entirely new mechanical joints capable of operating elastically. This elimination of traditional hinges and connectors could transform mechanical assemblies across various scales, enhancing performance while minimising material waste.
Despite these advancements, the path toward practical applications remains complex and necessitates further research and development. The team is committed to exploring not just the mechanical properties of these metamaterials, but also their long-term stability and performance under varying environmental conditions. Gaining a comprehensive understanding of how these materials behave in real-world scenarios is vital for moving their innovations into practical usage.
As the research progresses, interdisciplinary collaboration between mechanics, materials science, and engineering is emphasised as crucial for realising the full potential of these discoveries. The aim is to blend expertise from these fields to tackle the intricate challenges linked with the practical deployment of such advanced materials.
The implications of this research extend beyond material innovation; they also engage with broader environmental and economic issues surrounding sustainability and resource efficiency. With society’s increasing focus on reducing energy consumption while improving efficiency, advancements like these metamaterials are poised to represent significant progress. They not only promise enhancements to existing technologies but also cultivate possibilities for entirely new paradigms in energy storage and mechanical engineering.
The development of high-capacity mechanical metamaterials heralds a potential transformation in the understanding and utilisation of energy across diverse sectors. Applications spanning from robotics to energy-efficient machinery reflect a significant step toward sustainable practices that resonate with contemporary scientific and industrial challenges. As this research continues to unfold, it holds the promise not only of transforming technology but also of contributing significantly to overarching goals of sustainability and innovation.